ACS Nano Publishes Paper of ARO-supported Research on Miracle Organic-Inorganic Hybrid Semiconductors
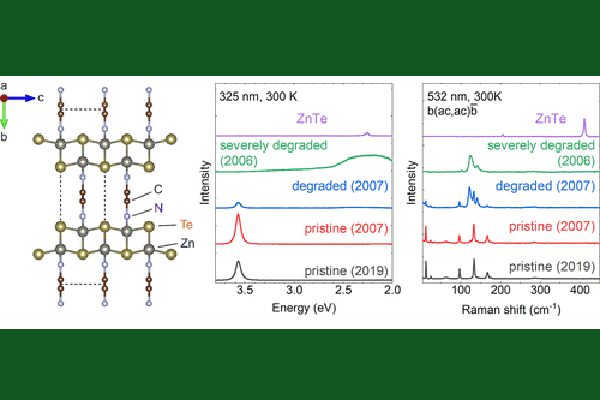
The American Chemical Society has published a paper on its website ACS Nano about the research of UNC Charlotte faculty members and their colleagues involving the development of a new miracle organic-inorganic hybrid semiconductor, which is one of the most stable hybrid and structurally most-perfect semiconductors known.
The research is sponsored by the Army Research Office (ARO), which is based in Research Triangle Park, NC, and is an element of the U.S. Army Combat Capabilities Development Command’s Army Research Laboratory. The name of the paper is “II−VI Organic−Inorganic Hybrid Nanostructures with Greatly Enhanced Optoelectronic Properties, Perfectly Ordered Structures, and Shelf Stability of Over 15 Years.”
A synopsis of the work is below:
Publication: “II−VI Organic−Inorganic Hybrid Nanostructures with Greatly Enhanced Optoelectronic Properties, Perfectly Ordered Structures, and Shelf Stability of Over 15 Years”. UNC Charlotte authors are Tang Ye, Margaret Kocherga, Andrei Nesmelov, Fan Zhang, Wanseok Oh, Damian Beasock, Daniel S. Jones, Thomas A. Schmedake and Yong Zhang. Non-UNC Charlotte authors are Yi-Yang Sun, Xiao-Ying Huang and Jing Li.
Support: ARO/Physical Properties of Materials (Grant No. W911NF-18-1-0079); University of North Carolina’s Research Opportunities Initiative (UNC ROI) through Center of Hybrid Materials Enabled Electronic Technology
Overview: People are interested in organic-inorganic hybrid materials, because they offer the advantages of the two worlds with either much improved or new properties compared to the organic and inorganic material alone. However, majority of known hybrid materials tend to have to drawbacks: (1) lower stability than the inorganic counterpart, and (2) structural disorder. The former often means that the hybrid material is more easily degraded under ambient condition or will quickly degrade with increasing temperature. The latter implies that the hybrid material typically lacks translational symmetry of a crystalline material, which in turn diminishes electrical conductivity and quantum coherence. The hybrid materials that have received the most interest in the past decade or so are a group of hybrid materials (e.g., MAPbI3, MA = CH3NH3) derived from halide semiconductors such as PbTe. They have shown great performance in solar cell and LED applications, but unfortunately, they also exhibit the two drawbacks, particularly the poor prospect in long-term stability. By long-term, we mean a time scale of one or two decades that is typically expected for (opto-)electronic applications.
The miracle organic-inorganic hybrid semiconductors are a group of II-VI based hybrid crystals that are miraculous in two senses: they have exceptionally long shelf life, over 15-years documented, compared to other hybrids; and yet they are structurally nearly perfect, even better than some well-known binary inorganic semiconductors.
A prototype example of these II-VI hybrids is ZnTe(en)0.5, where en (= C2N2H8) is an organic molecule called ethylenediamine. These materials were first reported around two-decade ago by Prof. Jing Li’s group at Rutgers University. They are ultra-thin layers of inorganic semiconductor sheets interconnected by organic molecules. Soon after the report, Prof. Yong Zhang, then at NREL, and his colleagues revealed a number of very unique properties, such as greatly enhanced exciton binding energy, unusually strong optical absorption and zero-thermal expansion. Recently, Zhang and Prof. Schmedake, supported by ARO, have re-initiated the study on these materials. Very surprisingly, they found some of the old samples studied over 15 years ago and kept under ambient condition remained practically the same as the newly made ones, although some were found degraded to various degrees. The finding suggests that the already long shelf life was still not limited by the material’s intrinsic, thermodynamic degradation mechanism, rather by other extrinsic mechanisms, such as surface imperfection or structural defects. Their thermal degradation study has yielded a projected room temperature half life over 10^8 years, an idealistic lifetime for a defect-free and properly encapsulated sample. By performing a first-principle density functional theory modeling, done by a collaborator, they have concluded that the intrinsic long-term stability is closely related to the formation energy of the hybrid structure. For ZnTe(en)0.5, the value is rather high due to covalent like chemical bonding between the inorganic and inorganic units (0.52 eV vs. 0.80 eV for ZnTe), whereas for the highly interested halide hybrid MAPbI3 the value is substantially smaller (0.1 eV). The degradation rate is expected to sensitively depend on the formation energy through an exponential function or a Boltzmann factor.
Further surprising finding is the observed unprecedent structural perfectness not only better than the known hybrid materials but also most inorganic semiconductors, such as GaAs and CdTe. The primary reason for the often-occurring structural disorder in the hybrid materials is the difficulty to assemble the large and low symmetry organic molecules together with the inorganic units into a highly organized structure. However, it does occur in the II-VI hybrids like ZnTe(en)0.5, despite its structural complexity with 32 or 64 atoms per nut cell, compared to binary compound like GaAs having only 2 atoms per unit cell. With much more complex structure, the X-ray diffraction linewidth of the II-VI hybrid was found only slightly larger than the best quality binaries like GaAs. However, in the microscopic scale, the hybrid is actually more perfect than the much simpler GaAs and CdTe where inevitable a significant amount of point defects is present even in the highest quality materials currently achievable. Because of the point defects, the internal quantum efficiency (IQE) of photoluminescence is much below 100 % at low excitation level, but the ZnTe(en)0.5 has been shown to have nearly 100% at the comparable excitation level, which is even better than the best reported hybrid. Therefore, it could be viewed as the structurally most perfect semiconductor.
These hybrid structures are special in another aspect – as a most perfect man-made semiconductor superlattice. A superlattice refers to a structure with alternating layers of material A and B stacked in a periodic manner, first proposed by Esaki and Tsu in 1970. However, after 5 decades, people are still struggling to achieve abrupt stacking interfaces, because no matter what material A and B tend to inter-diffuse into each other, which becomes more problematic when the layers are down to a few monolayer thick. Monolayer is the thinnest unit of the material. Here the hybrid structure like ZnTe(en)0.5 can be viewed as a hybrid superlattice with two monolayer ZnTe and one monolayer of organic molecule. The structural perfectness of the hybrid makes it possible to investigate a number of physical properties of the superlattice that have been obscured by the inevitable interfacial disorder in all the known inorganic superlattices, for instance, the vibration modes of the superlattice.
These II-VI hybrid materials tend to have large electronic bandgaps and, consequently, are appropriate for applications in UV detection and emitting devices, transparent transistors, sensors, conductive layers, and electronic or hole transport layers in optoelectronic devices. Because of their large exciton binding energies, resulting from quantum confinement and reduced screening in low dimensions, these hybrid materials are also promising candidates for room temperature exciton-polariton condensation and related quantum effects.
For more information contact Dr. Yong Zhang at Yong.Zhang@uncc.edu